Tech Article
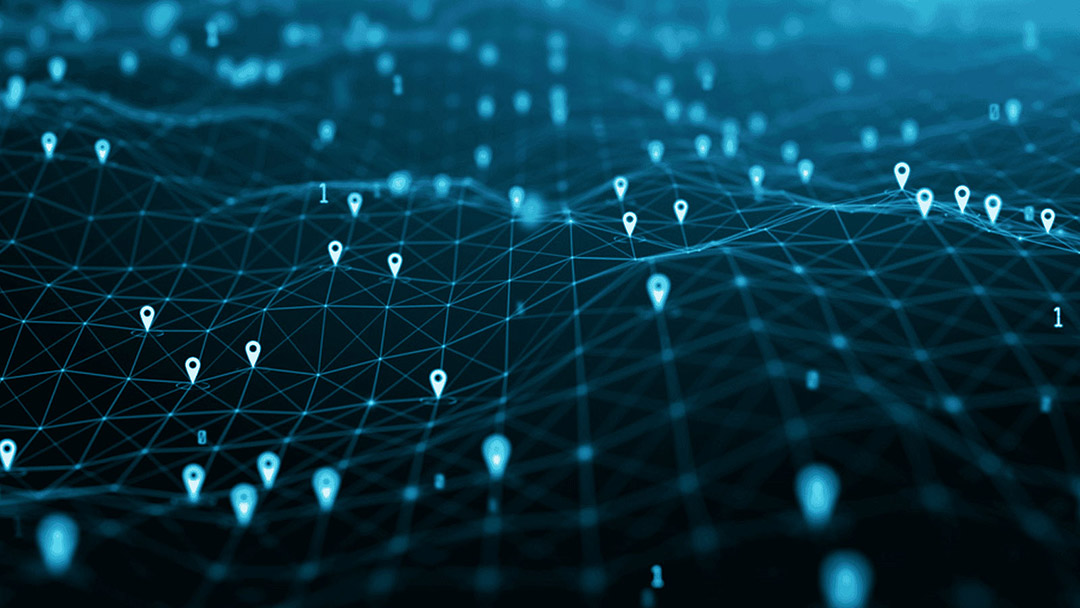
Published on:
Before we take a look into post-processing kinematics software, let’s define the term “kinematics”. Basically, kinematics is the study purely of the motion of objects, without concern for the forces involved causing the motion.
In a navigational sense, kinematics is a bit different to the definition above. We are still wanting to gain motion information for a moving object, but the context here is to establish the true path (or as close to the truth as we can get) of the object in relation to an absolute position on the surface of the Earth.
The global navigation satellite system (GNSS) provides our equipment with data that is used to establish an absolute position, with a certain degree of accuracy. To summarize, each satellite in the various GNSS constellations repeatedly sends signals toward the Earth that basically identify the satellite and provides its orbital position and trajectory data, known as ephemeris data, and the time that the signal was sent. There may be additional data; for example, any known orbit error. GNSS receivers on the roving device pick-up these signals and, using the time differential between when the signal was sent and received, can estimate the distance between the rover and satellite. When four or more different satellites can be “seen” by the receiver at the same time, a position that includes altitude can be calculated.
There are several GNSS satellite constellations orbiting the Earth. Each providing data for positioning in various continental areas
The important thing to note is that the satellite signals and resulting position calculations alone do not provide reliable, near pinpoint accuracy. This is where additional hardware, mathematics and techniques are used – to correct errors that accumulate by the time the satellite signals are received on Earth.
In a word, accuracy, which can be defined as the difference between a navigation solution and the truth. The standard method of obtaining position on a revolving Earth using radio signals from a few orbiting satellites located 20000 km away is quite amazing in that position is accurate to a radius of approximately 5 m. This might be acceptable for non-critical applications, such as basic car sat-nav systems and mobile phone location, however, for applications that rely on very precise bearings, down to as little as 1 cm, a great deal more accuracy is needed.
There are several phenomenon that can introduce GNSS errors:
Error Type: Ionospheric
Range: ± 5 m
Signal propagation delay – upper atmosphere is loaded with electrons caused by ionizing solar radiation that can “bend” and reflect radio waves.
Error Type: Orbit
Range: ± 2.5 m
Position drift – as with clocks, minuscule errors in satellite orbit position become much larger when used for position calculation on Earth.
Error Type: Clock
Range: ± 2 m
Timing drift – due to the distances, tiny timing errors in satellite clock accuracy become much larger errors on Earth.
Error Type: Multipath
Range: ± 1 m
Signal replication due to reflection off objects such as buildings and terrain.
Error Type: Tropospheric
Range: ± 0.5 m
Signal propagation delay – lower atmosphere is far denser than other atmospheric layers and can refract radio waves.
Error Type: Receiver noise
Range: ± 0.3 m
GNSS receiver hardware and software induced signal noise that affects accuracy of perceived signal.
The individual errors appear relatively minor when you consider the huge distances involved between satellites and the Earth. When these errors are combined, they can result in substantial losses in accuracy that are not acceptable in many applications, such as commercial aircraft and marine navigation systems and survey grade applications.
All across the globe, there are large numbers of base stations that are installed as on-Earth references for GNSS error correction purposes. Base stations are a critical part of GNSS infrastructure in terms of signal correction and overall improvement in system accuracy and reliability. The base station locations are known to a very high degree of accuracy, so the base station itself becomes a referenceable location.
The base station receives GNSS signals and uses carrier wave measurements to precisely calculate distances to observable satellites. That is, by using the signal wavelength multiplied by the number of cycles, plus the signal phase position of the final waveform. In addition, the ephemeris data included in the satellite signals is used to help calculate GNSS signal errors; for example, calculating ionospheric delays.
GNSS signal pseudorange, carrier phase, signal to noise ratio and Doppler measurements made by the base station are constantly logged and archived. The logs are sent to servers, which then provide the data in RINEX file format. RINEX files can be downloaded and used by post-processing kinematic solutions. For real-time solutions, the base station provides satellite observations in real-time. This data can be transmitted to users via terrestrial radio links, cellphones and the Internet. Roving navigational devices obtain and apply the necessary corrections to determine a more accurate location for the device.
To overcome inaccuracies, there are a number of techniques that are designed to deduce errors and remove them from position calculations. Most rely on real-time base station connections. Note that RTK, which can be accurate to 1 cm in ideal conditions, is perhaps the most widely used technology. The following are some examples:
Real-time solutions rely on solid data connections not only to satellites but also to base stations and/or the internet or additional satellite services. This makes them prone to being affected by connection interruptions as well as the possibility of small estimation errors for some GNSS signal variables. Furthermore, proximity to base stations in itself introduces an additional error the further the roving receivers are from the base station – best results are obtained when under 50 km from a base station.
PPK software or online services are capable of processing uncorrected navigational data and achieving equivalent or even better than RTK accuracy – down to sub 1 cm. Importantly, PPK is suited to applications that do not have to rely on real-time corrections. For example, a UAV surveying mission can easily be post-processed, however, an autonomous tractor in a field requires real-time corrections. Not only that, but solutions such as RTK may require other considerations, such as higher initial cost, licensing for correction services, more hardware and require more on-board processing power. For applications that are weight or payload sensitive, such as aerial surveying using UAV LiDAR, the additional weight and power requirements may impact flight duration and overall cost or durability of the solution.
Post-processed and real-time position correction solutions both process the same types of data, including observations of solar radiation pressure on satellites, satellite ephemeris information (including position, velocity and clock bias), inertial sensor measurements from the roving navigation device (if applicable) and inputs from other navigational aids, such as an odometer. PPK, however, will typically also use data collected before and after the event during analysis and processing, making for a superior solution.
The main difference between post-processing and real-time navigation correction solutions is the source of the satellite ephemeris data and, of course, when the corrections are applied. A post-processing solution, such as Advanced Navigation Kinematica PPK software, typically downloads historical ephemeris and base station correction data from online archives. Additionally, data from multiple satellites and base stations can also be included in the processing. The major advantage of this is that the accumulation of ephemeris and correction data over time and from multiple sources provides a higher level of accuracy over real-time solutions. Note that for best PPK results, it is recommended to allow 24 hours to pass before post-processing. This allows for a greater accumulation of satellite ephemeris and correction data that can be downloaded and used in processing.
Some PPK software solutions are able to not only process raw GNSS data, but also INS or IMU data. This is especially useful in situations where GNSS becomes degraded or is lost. For example, when road surveying through tunnels or urban canyons. During this time, dead-reckoned navigational data from the INS is the only input. A post-processing kinematics solution may process mission data multiple times in both a forward and reverse direction. Using a process of comparison between each pass, the system is able to identify and reduce drift errors to deliver a smoother, more accurate result in comparison to a real-time solution. This is especially useful between points where GNSS was lost and refining and correcting the dead-reckoned data.
Example image showing GNSS satellite signals (red lines) to base station (left) and rover (centre). Corrected satellite data (green lines) is broadcast by base station for real-time solutions and archived for post-processing solutions. PPK solution (right) analyzes and processes archived correction data and GNSS data from mission (blue lines)
Post-processing is appropriate for a wide variety of applications, where navigation determinations (position, velocity, heading etc) do not have to be made at the time that the data is being generated. Typical examples of applications that can benefit from post-processing using PPK software include:
Kinematica post processing kinematics software offers the following benefits to provide additional convenience and to consistently achieve a high degree of accuracy:
20 May 2025
Go to Article30 March 2025
Go to Article